Article de Loïs Miraux (EnvIM 2021)
This article is a shortened and adapted version of a paper from the author published in Science of the Total Environment, available at: https://www.sciencedirect.com/science/article/pii/S0048969721059404#
1. Introduction
The scale of anthropogenic actions on the Earth system has become so large that they are now the main driving force of environmental change, leading to the rise of a new geological era, the Anthropocene1. As a result, the stable environmental conditions that allowed human civilizations to rise and thrive are now under threat because of human activities. Undermining this stability could shift Earth subsystems into new states dangerous for humans. This means that there are biophysical limits that humans need to respect to keep Earth a “safe place”, as expressed by the planetary boundaries framework2. Our highly unsustainable contemporary societies are, therefore, facing the daunting challenge of rapidly transitioning towards a system operating within these limits while meeting fundamental human needs.
In this context, satellites provide key services to society including progress in space and Earth science, telecommunication, navigation, surveillance, and are particularly useful for environment and resource management. For instance, they provided major advances in climate science3, monitor more than half of the 50 Essential Climate Variables, and are essential to inform decision-making for mitigation and adaptation4. This means that the sustainability of space activities is important and must be guaranteed.
However, the space sector is undergoing profound transformations, shifting from the “traditional space” driven by government investments to the “NewSpace”, primarily driven by commercial motivations: in 2019, commercial activities represented 79% of the global space economy5. This shift has been enabled by technological and business model innovations including advances in manufacturing, miniaturization, and reusable launch systems, leading to a significant reduction in cost and the appearance of new products and services6. As a result, the global space economy grew by 6.3% per year on average between 2009 and 2019, reaching a total value of 423.8bn$ in 2019 [7], and is expected to reach 2.7tn$ by 20457. Upcoming projects include constellations consisting of thousands of satellites, reusable rockets, space tourism (suborbital flights, space flights, space hotels), but also more ambitious endeavors such as Moon bases, Mars colonization, rocket Earth-to-Earth transportation, asteroid mining, or space-based solar power (Fig.1).
Space activities are, therefore, on the verge of a great increase. But like all human activities, they have impacts on the environment. This article reviews the most critical impacts of the space sector as well as their potential growth, analyzed together in a comprehensive approach. Pollution from objects in space (space debris and night sky pollution) is described and the use of a framework based on planetary boundaries is proposed as a way to express its limits. Then, atmospheric impacts and associated regulatory risks are detailed. Limits to the development of space activities emerging from these environmental impacts are outlined, and the relevance of their consideration by actors in the space sector is emphasized. Finally, the future of the environmental, economic, and social sustainability of the space sector in the context of global ecological transition is discussed.
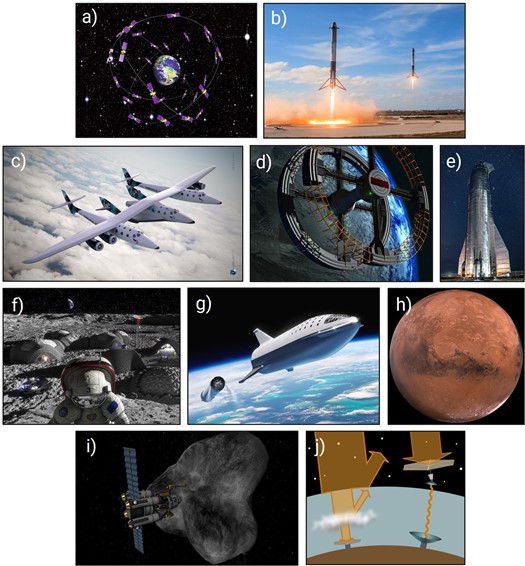
2. Limits due to pollution on orbit
The number of objects in orbit – large enough to be traceable – has increased steadily since the beginning of the space age and has nearly doubled in the last decade25. About 85% of these traceable objects are abandoned spacecraft or fragmentation debris from explosions or collisions. However, the vast majority of debris is untraceable and their number estimated by statistical analysis is 128 million objects26. The creation of debris increases the exposure of other satellites to the risk of collision that can lead to malfunctions or even complete disintegration, and thus constitutes a degradation of the orbital resource. Since the critical density of objects beyond which cascading debris collisions are self-sustaining has already been reached in some orbital regions, the number of objects in orbit will continue to increase even in the absence of additional launches over hundreds or thousands of years25. The loss of some orbital regions due to space debris would have an impact on the entire economy because it could permanently disable essential services that modern society relies on, such as GPS navigation, communications, early warning systems, and weather and environmental monitoring services. In this context, while only about 2000 satellites were in orbit in 2018, recent plans to launch satellite constellations amounting to more than 20,000 satellites by 2030, and possibly more than 60,000 over the following decade, are raising growing concerns.
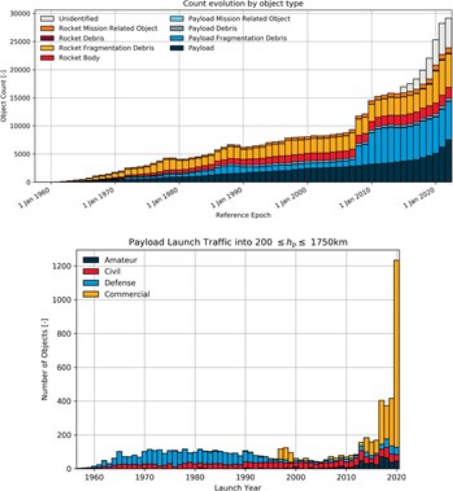
In addition to endangering the sustainable use of the space environment, large constellations can, by reflecting sunlight back to Earth and emitting radio signals, negatively impact the visibility of the night sky and interfere with professional astronomical observations27. The overall effect of satellites and space debris on light pollution has been estimated by a recent study. They would be responsible for a diffuse skyglow effect already increasing the luminosity of the night sky of 10 % over natural levels28. This is above the threshold set by the international astronomy community defining an astronomical site as polluted.
Space debris is a major threat to the long-term sustainability of space activities. The pristine night sky is a common heritage of humankind: the discussed changes may have, in addition to impacts on stargazing and scientific inquiry, unforeseen effects on wildlife, human health, and cultural and religious practices. Without further precaution, the unregulated action of private interests in space could lead to the tragedy of two commons — the near-Earth orbital environment and the night sky — which must be avoided. In this context, the formulation of new boundaries for the proliferation of objects in orbit and night sky brightness could be adopted to make use of the widely adopted concept of planetary boundaries to encourage sustainable action and avoid or limit their transgression.
3. Impacts on the Earth’s atmosphere: is the sky the limit?
The mass of material emitted by the 100 rockets launched every year represents only 0.01% of the fuel burned by the aviation sector29. However, as they ascend from the ground to orbit, they release gases and particles into all layers of the atmosphere. Emissions in the stratosphere, the layer above the troposphere, are of greatest concern because emissions from hundreds of launches accumulate there over several years, and because this is where the ozone layer is located.
During the lifecycle of complete space missions, the launch event has been reported to contribute to almost 100% of the ozone depletion potential30. The ozone loss caused by the global launch fleet has been estimated to be more than 0.01% and less than 0.1% of the total amount of ozone31. This compares to the ozone loss caused by ozone-depleting substances (ODS) banned by the Montreal Protocol of about 3%.
While the effect of rocket emissions on the ozone layer has been studied for several decades, the concern about their impact on climate is more recent. Available life cycle assessment studies of space missions are scarce and often do not account for emissions occurring during the launch event, or only partially, due to lack of data availability and modeling complexity30,32–34. Yet, launch emissions are likely to be the most important contributor to the impact on climate change of the global space sector. The amount of greenhouse gases emitted is insignificant, but the effect of the particles (alumina, black carbon) emitted by certain types of fuels is more concerning. By accumulating in the stratosphere and absorbing a fraction of sunlight, they cause a warming of this layer (Fig.3). In 2014, rocket emissions were estimated to contribute globally to warming the stratosphere by about 16±8 mW/m², which could lead to a global cooling of the troposphere with local warming risks29,35. Rocket launches would, therefore, be uncontrolled geoengineering micro-experiments36. By comparison, the radiative forcing of global aviation on the troposphere is only higher by a factor of 4 for about 35 million annual flights29.
Therefore, the current contribution of rockets to ozone loss and climate change remains small, although extreme considering the small number of launches. However, the emerging markets mentioned in the introduction could lead to a 10-fold increase in launcher emissions in the coming decades, which would make their contribution to ozone depletion comparable to that of ODS and their contribution to radiative forcing greater than that of global aviation. For these reasons, there will be a growing risk that the launch industry attracts regulatory attention.
![Figure 3 - Effects of rocket particle accumulation in Earth’s atmosphere: stratospheric injection of particles destroys ozone, but also warms the stratosphere, resulting in a global cooling of the troposphere and circulation changes that can result in regional warming (locations in the figure are purely indicative). Inspired from: The Policy and Science of Rocket Emissions, The Aerospace Corporation [64].](https://blog-isige.minesparis.psl.eu/wp-content/uploads/2022/01/Image4.jpg)
Furthermore, due to natural orbital decay or voluntary deorbiting maneuvers, objects in Low Earth Orbit (LEO) fall back down to Earth and burn up when reentering the atmosphere. However, particles originating from these burning spacecraft (e.g. aluminium) could have detrimental effects on the ozone layer or on climate. Particles of several metals resulting from thousands of large constellations’ satellites reentering would exceed by far injection of natural origin such as meteorites, but the resulting effect is yet unknown37. Although purely hypothetical at this stage, it is possible that at a critical rate of reentering objects, the resulting pollution on the atmosphere reaches a level raising regulatory attention from policymakers. This could limit the rate of object disposal in LEO, thereby limiting the rate at which new objects could be launched there because the orbital resource is limited by the risk of space debris (Fig.4).
4. Environmental sustainability and beyond
Consideration of environmental limits
It is generally assumed that the only limitations to the development of the space sector are either technological or economic. However, the previous discussion shows that there are also environmental limitations that can arise either from (Fig.4):
- Transgression of the boundary of the proliferation of objects in orbit and/or associated regulations
- Transgression of the boundary of light pollution at night and/or associated regulations
- Regulations on climate radiative forcing and ozone depletion from launchers emissions
- Regulations on climate radiative forcing and ozone depletion from spacecraft reentry emissions
These limits are intertwined. A satellite disintegrated into debris is likely to have a higher contribution to night sky brightness than its intact version because of increased reflecting surface area, implying that space debris proliferation could aggravate night sky pollution. In addition, a recent study found that ozone destruction leads to a degradation in plants’ capabilities to store carbon because of damages from UV radiation38. Impacts of rockets on ozone, therefore, indirectly increase their contribution to climate change. In these cases, this also means that measures to mitigate environmental impacts can have co-benefits. On the contrary, space debris and the atmospheric impacts of reentry have conflicting mitigation measures.
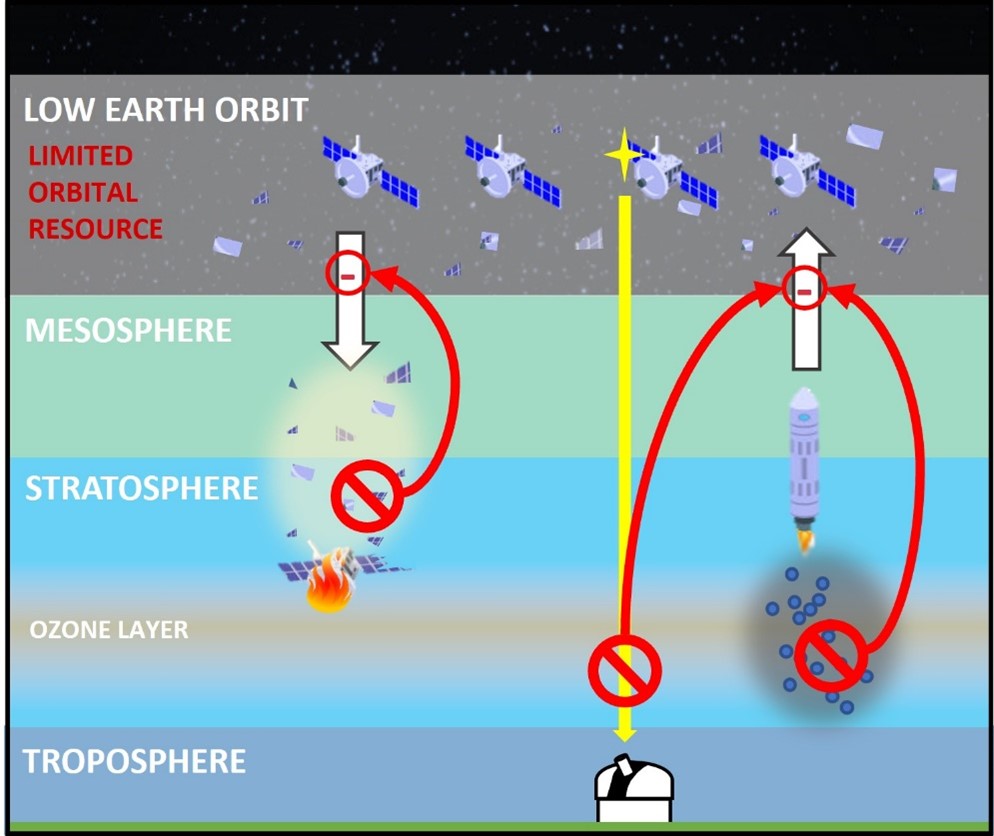
Sustainability assessments and ecodesign of space systems
The consideration of environmental issues in the space sector is very recent, but there is a growing interest in assessing and mitigating the impacts of space activities. The European Space Agency is leading the development of Life Cycle Assessment (LCA) and ecodesign practices with its Clean Space initiative started in 2012. LCA in space applications presents various specific challenges, such as the difficulty of data collection and the need for specific data sets due to the use of specialty materials, advanced manufacturing processes, and small production volumes33,39 . In addition, space activities can pollute in unique, sector-specific ways requiring extensions of the LCA scope. A framework for assessing impacts on space debris within LCA was recently developed40 and applied on an existing mission41. Some authors have even considered extensions of the LCA scope for space exploration and space travel42. While this is an interesting exercise, more pressing, new concerns on night sky pollution could make the inclusion of this parameter also relevant, especially for large constellations.
A first attempt to assess the sustainability of the global space sector was carried out by A.Wilson in his PhD thesis34, using a streamlined Life Cycle Sustainability Assessment evaluating environmental, social, and economic impacts. The approach consists of an approximation of these impacts over one year, which are compared to 2010 worldwide impacts and planetary boundaries. In a future scenario assuming 750 launches per year delivering 5000 spacecraft into orbit, the contribution of the space sector to climate change was found to reach 1.77% of the associated planetary boundary, and 1.54% for ozone depletion. These results are already significant considering the small relative size of the space sector with respect to all human activities. Moreover, it is important to note that despite being a major source of concern, the effects of black carbon and alumina on climate change and ozone depletion were not characterized due to knowledge gaps. Instead, black carbon was not considered in rockets’ exhaust compositions and alumina was reported as a flow indicator. In addition, the impacts of other emissions were calculated using characterization factors that were not altitude dependent, again due to knowledge gaps, meaning that emissions in the troposphere and in the stratosphere had the same effects. It is therefore urgent to bridge these gaps to enable sustainability assessments accounting for these most critical impacts. This is a necessary condition to be able to make recommendations to the industry on how to design cleaner launch systems.
Ecodesign practices are particularly relevant for the space sector which is characterized by stringent safety and reliability requirements and very long lifecycles from design to exploitation phases. Decisions that can be critical for the environmental performance of a space system are made in the early development phase43 and later changes are often impossible after the qualification of the design. Development cycles of launchers usually vary between 5 and 10 years, while the exploitation time is as long as possible to recover development costs. For instance, Ariane 5 made its maiden flight in 1996 and is still flying today. This means that launchers that are currently under development may still be operating in 2050.
This causes several risks for the space sector. In the context of the European REACH regulations (Registration, Evaluation and Authorisation of Chemicals), obsolescence risks were identified for the space industry44, which would undermine the quality, reliability, or even the feasibility of a technology. Regulatory obsolescence can arise from a legal ban on chemical substances, while commercial obsolescence can occur when suppliers change a product used by the space industry because larger sectors stop using it. It was reported that 20% of the materials used in the space industry may be affected in the long term44. These risks related to REACH are insightful for those that can be caused by the environmental limits previously outlined: for instance, a regulation on stratospheric injection of black carbon could make a launch system obsolete well before its planned date of end of exploitation, resulting in significant losses for the operator.
Space activities in times of environmental breakdown
Although space missions have contributed and will continue to contribute significantly to address environmental issues, many incoming projects are out of step with – or even in contradiction with – these critical issues. Megaconstellations offer ubiquitous access to high-speed internet services, fueling the growth of the digital sector whose energy and material needs are becoming unsustainable. Space tourism, which is rapidly emerging, is perhaps the most powerful demonstration of issues related to environmental justice. At the time of the “flygskam”, SpaceX proposes to develop an ultra-fast commercial Earth-to-Earth transport system by rocket. Other projects are put forward as potential solutions to environmental and material problems on Earth, such as generating electricity with orbital solar stations, mining asteroids, and even reaching Mars with the goal of making humanity a multi-planetary species by colonizing a “Planet B.” However, these solutions have little credibility given the short time interval between the collision of the current development model and planetary limits, and continue to feed the vain belief of a technological salvation. For instance, the pioneer Chinese space-based solar power plant is planned to reach 1GW by 2050, which over a year would produce only 0.1% of its 2018 electricity needs, expected to grow by 205045. This will be a meager contribution to China’s carbon neutrality planned for 2060, not to mention the plan to phase out coal by 2040 as required for 1.5°C compatible pathways46. On the other hand, as the mineral requirements for clean energy technologies would increase by a factor of 6 by 2040 to reach global carbon neutrality by 205047, space mining is not credible as a potential solution in this context.
Fast and continuous growth of space activities over the next decades, fueled by the various projects described in the introduction, could pose serious threats to both the space and Earth environments. However, in the same period, the global economy, energy systems, and social structures will undergo profound transformations due to the global environmental threats of climate change, biodiversity loss, freshwater scarcity, resource depletion and various forms of pollution. Responding effectively to these challenges will very likely constrain economic growth, given that the green growth paradigm — the decoupling of economic growth from environmental pressures — lacks empirical evidence, is highly unlikely to be achieved rapidly enough to meet climate targets and is unlikely to happen at all48–50. This will also create tensions on some resources, as many studies have reported a dramatic increase in material requirements for a transition to a low-carbon society47,51–56 with some materials having high levels of criticality57,58.
In this context, in addition to their potentially severe impacts on the environment, the significant economic, energetic, and material requirements of some proposed space projects like space colonization and Earth-to-Earth transportation could be prohibitive. They could also become increasingly socially unacceptable and ethically questionable. Given the importance of the services provided to human societies, the question of guaranteeing access to space during the Anthropocene transition is crucial. This also raises the question of the vulnerability induced by systemic dependence on space services. On a more distant horizon, the very survivability of the space industry can be questioned considering the probable future diminution of the global energy return on investment (EROI) and its link with the level of advancement of societies59.
As a result, the space industry, which relies on high social, industrial, and technological complexity, international supply chains, and critical materials60, may see its predicted growth, planned agenda, and established politico-economic support seriously challenged in the next decades. Given the long design and lifecycle timescales of space projects, the space sector’s decision-makers should also consider these constraints when making plans for the future, in addition to the environmental limitations previously outlined.
Considering the environmental limits to the space sector’s growth discussed, the trends of fast growth, commercialization, and search for maximization of profit that are fueling the NewSpace must not undermine space activities that are unambiguously beneficial for mankind. In addition, beyond considerations on the applications of space technologies, the existence of environmental limits constraining the development of space activities has important implications for the future expansion of mankind in space. The visions of space as a new frontier that mankind will inevitably conquer and of a future in which humanity becomes a multi-planetary species are challenged by the physical reality of planetary and orbital boundaries: we may find that shooting for the stars comes at an unbearable cost for the Earth’s environment.
–
References
- Crutzen, P. J. The “Anthropocene”. in Earth System Science in the Anthropocene (eds. Ehlers, E. & Krafft, T.) 13–18 (Springer, 2006). doi:10.1007/3-540-26590-2_3.
- Rockström, J. et al. A safe operating space for humanity. Nature 461, 472–475 (2009).
- Yang, J. et al. The role of satellite remote sensing in climate change studies. Nature Climate Change 3, 875–883 (2013).
- CEOS. The CEOS Earth Observation Handbook, Special 2015 COP21 Edition: Satellite earth observations in support of climate information challenges. http://eohandbook.com/cop21/.
- Global Space Economy Grows in 2019 to $423.8 Billion, The Space Report 2020 Q2 Analysis Shows. Space Foundation https://www.spacefoundation.org/2020/07/30/global-space-economy-grows-in-2019-to-423-8-billion-the-space-report-2020-q2-analysis-shows/ (2020).
- European Investment Bank. The future of the European space sector. 162 (2019).
- Merrill-Lynch sees big growth long-term for space industry. NewSpace Global https://newspaceglobal.com/merrill-lynch-sees-big-growth-long-term-space-industry/ (2017).
- Curzi, G., Modenini, D. & Tortora, P. Large Constellations of Small Satellites: A Survey of Near Future Challenges and Missions. Aerospace 7, 133 (2020).
- DLR, G. A. C. Artist’s impression of the Galileo constellation. (2014).
- Official SpaceX Photos. Falcon Heavy Demo Mission. (2018).
- Wigley, T. Virgin Galactic’s Mothership and SpaceShip Two. (2008).
- Voyager Station. https://voyagerstation.com/.
- Free photo Space Station Space Travel Planet Earth Atmosphere – Max Pixel. https://www.maxpixel.net/Space-Station-Space-Travel-Planet-Earth-Atmosphere-423702.
- Elon Musk Says SpaceX’s Giant Mars Rocket Could Fly Passengers Around Earth. Space.com https://www.space.com/38314-elon-musk-spacex-mars-rocket-earth-travel.html.
- Official SpaceX Photos. Starship | First test vehicle. (2019).
- NASA: Artemis. NASA https://www.nasa.gov/specials/artemis/index.html.
- Group, 2018, E. R., visualisation: Liquifer Systems. English: A vision of a future Moon base that could be produced and maintained using 3D printing. (2018).
- Corp, S. E. T. English: The 2018 version of the Big Falcon Rocket at stage separation: Starship (foreground) and Super Heavy (background). (2018).
- TESMANIAN. SpaceX plans to send a fleet of 1,000 Starships to Mars. TESMANIAN https://www.tesmanian.com/blogs/tesmanian-blog/mars-city.
- Image gratuite sur Pixabay – Mars, Planète Rouge, Planète. https://pixabay.com/fr/photos/mars-plan%C3%A8te-rouge-plan%C3%A8te-espace-11012/.
- ASTRA : Asteroid Mining Technologies Roadmap and Applications. (International Space University, 2010).
- Kordite. Miner Fourty-Niner. (2015).
- China’s super heavy rocket to construct space-based solar power station. SpaceNews https://spacenews.com/chinas-super-heavy-rocket-to-construct-space-based-solar-power-station/ (2021).
- Chabacano. Español: A la izquierda, al atravesar los rayos solares la atmósfera se pierde energía debido a la absorción o refexión. A la derecha, la energía solar espacial pretende capturar la energía solar fuera de la atmósfera y transmitirla a la superficie. (2008).
- European Space Agency. ESA’s Annual Space Environment Report 2021. https://www.sdo.esoc.esa.int/environment_report/Space_Environment_Report_latest.pdf.
- Space Environment Statistics · Space Debris User Portal. https://sdup.esoc.esa.int/discosweb/statistics/.
- International Astronomical Union, IAU. IAU Statement on Satellite Constellations. https://www.iau.org/news/announcements/detail/ann19035/.
- Kocifaj, M., Kundracik, F., Barentine, J. C. & Bará, S. The proliferation of space objects is a rapidly increasing source of artificial night sky brightness. Monthly Notices of the Royal Astronomical Society: Letters 504, L40–L44 (2021).
- Ross, M. N. & Sheaffer, P. M. Radiative forcing caused by rocket engine emissions. Earth’s Future 2, 177–196 (2014).
- Chanoine, A. Environmental impacts of launchers and space missions. 36 (2017).
- Ross, M., Toohey, D., Peinemann, M. & Ross, P. Limits on the Space Launch Market Related to Stratospheric Ozone Depletion. Astropolitics 7, 50–82 (2009).
- Gallice, A. Environmental Impact of the Exploitation of the Ariane 6 Launcher System. (2020).
- Maury, T., Loubet, P., Serrano, S. M., Gallice, A. & Sonnemann, G. Application of environmental life cycle assessment (LCA) within the space sector: A state of the art. Acta Astronautica 170, 122–135 (2020).
- Wilson, A. Advanced Methods of Life Cycle Assessment for Space Systems. (2019).
- The Coming Surge of Rocket Emissions. Eos https://eos.org/features/the-coming-surge-of-rocket-emissions.
- The Aerospace Corporation. The Policy and Science of Rocket Emissions. https://aerospace.org/sites/default/files/2018-05/RocketEmissions_0.pdf.
- Schulz, L. & Glassmeier, K.-H. On the anthropogenic and natural injection of matter into Earth’s atmosphere. Advances in Space Research 67, 1002–1025 (2021).
- Young, P. J. et al. The Montreal Protocol protects the terrestrial carbon sink. Nature 596, 384–388 (2021).
- Pettersen, J. B. & Viak, A. Ecodesign for space and aerospace: what happens when we make ecodesign relevant for demanding applications? 14 (2015).
- Maury, T. et al. Assessing the impact of space debris on orbital resource in life cycle assessment: A proposed method and case study. Science of The Total Environment 667, 780–791 (2019).
- Maury, T., Morales Serrano, S., Loubet, P., Sonnemann, G. & Colombo, C. Space debris through the prism of the environmental performance of space systems: the case of Sentinel-3 redesigned mission. Journal of Space Safety Engineering 7, 198–205 (2020).
- Ko, N., Betten, T., Schestak, I. & Gantner, J. LCA in space − current status and future development. Matériaux & Techniques 105, 507 (2017).
- Chanoine, A. Integrating sustainability in the design of space activities: development of eco-design tools for space projects. 11 (2015).
- AD Aerospace. REACH REFIT 2017 Position Paper. https://eurospace.org/wp-content/uploads/2018/09/eurospace-position-paper-reach-refit-2017-27jan2017.pdf.
- China – Countries & Regions. IEA https://www.iea.org/countries/china.
- China. https://climateactiontracker.org/countries/china/.
- Executive summary – The Role of Critical Minerals in Clean Energy Transitions – Analysis. IEA https://www.iea.org/reports/the-role-of-critical-minerals-in-clean-energy-transitions/executive-summary.
- Growth without economic growth — European Environment Agency. https://www.eea.europa.eu/publications/growth-without-economic-growth.
- Parrique, T., Barth, J., Briens, F., Spangenberg, J. & Kraus-Polk, A. Decoupling Debunked. Evidence and arguments against green growth as a sole strategy for sustainability. A study edited by the European Environment Bureau EEB. (2019).
- Wiedmann, T., Lenzen, M., Keyßer, L. T. & Steinberger, J. K. Scientists’ warning on affluence. Nature Communications 11, 3107 (2020).
- Chatterjee, S. & Huang, K.-W. Unrealistic energy and materials requirement for direct air capture in deep mitigation pathways. Nature Communications 11, 3287 (2020).
- Giurco, D., Dominish, E., Florin, N., Watari, T. & McLellan, B. Requirements for Minerals and Metals for 100% Renewable Scenarios. in Achieving the Paris Climate Agreement Goals: Global and Regional 100% Renewable Energy Scenarios with Non-energy GHG Pathways for +1.5°C and +2°C (ed. Teske, S.) 437–457 (Springer International Publishing, 2019). doi:10.1007/978-3-030-05843-2_11.
- Moreau, V., Dos Reis, P. & Vuille, F. Enough Metals? Resource Constraints to Supply a Fully Renewable Energy System. Resources 8, 29 (2019).
- Sovacool, B. K. et al. Sustainable minerals and metals for a low-carbon future. Science 367, 30–33 (2020).
- Vidal, O., Goffé, B. & Arndt, N. Metals for a low-carbon society. Nature Geoscience 6, 894–896 (2013).
- Watari, T. et al. Total material requirement for the global energy transition to 2050: A focus on transport and electricity. Resources, Conservation and Recycling 148, 91–103 (2019).
- European Commission. Joint Research Centre. Institute for Energy and Transport., Oakdene Hollins Ltd., & Fraunhofer Institute for Systems and Innovation Research ISI. Critical metals in the path towards the decarbonisation of the EU energy sector :assessing rare metals as supply chain bottlenecks in low carbon energy technologies. (Publications Office, 2013).
- Graedel, T. E., Harper, E. M., Nassar, N. T., Nuss, P. & Reck, B. K. Criticality of metals and metalloids. Proc Natl Acad Sci USA 112, 4257–4262 (2015).
- Lambert, J., Hall, C. & Balogh, S. EROI of Global Energy Resources: Status, Trends and Social Implications. (2013). doi:10.13140/2.1.2419.8724.
- Pavel, C. C. & Tzimas, E. Raw materials in the European defence industry. 126.